一次LINUX网络协议栈的探根之旅
公司的公共库中发现一段代码,简单化之,如下
...
struct sockaddr_un srv_sun;
int fd = socket(AF_UNIX, SOCK_STREAM, 0);
srv_sun.sun_family = AF_UNIX;
strcpy(srv_sun.sun_path,"/tmp/something_socket");
int srv_len = sizeof(srv_sun);
int ret = bind(fd, (struct sockaddr *)&srv_sun, srv_len);
...
我们知道sockaddr
和sockaddr_un
的结构不一样。按照UNP的写法:
struct sockaddr{
uint8_t sa_len;
sa_family_t sa_family;
char sa_data[14];
};
struct sockaddr_un{
uint8_t sun_len;
sa_family_t sun_family;
char sun_path[104];
};
疑惑1
那么问题来了,strcpy
后,srv_sa
的sun_path
长度明显超过sockaddr
的sa_data
的长度,那么bind
强制转换后是否有问题?
因此就想查一下bind
函数的实现是什么样的,辗转反侧,最终没有找到直接的bind
函数实现,但是找到了下面的东西。位于xxx/linux/kernels/mips-linux-2.6.31/net/socket.c
。
Location: xxx/linux/kernels/mips-linux-2.6.31/net/socket.c
/*
* Bind a name to a socket. Nothing much to do here since it's
* the protocol's responsibility to handle the local address.
*
* We move the socket address to kernel space before we call
* the protocol layer (having also checked the address is ok).
*/
SYSCALL_DEFINE3(bind, int, fd, struct sockaddr __user *, umyaddr, int, addrlen)
{
struct socket *sock;
struct sockaddr_storage address;
int err, fput_needed;
sock = sockfd_lookup_light(fd, &err, &fput_needed);
if (sock) {
err = move_addr_to_kernel(umyaddr, addrlen, (struct sockaddr *)&address);
if (err >= 0) {
err = security_socket_bind(sock,
(struct sockaddr *)&address,
addrlen);
if (!err)
err = sock->ops->bind(sock,
(struct sockaddr *)
&address, addrlen);
}
fput_light(sock->file, fput_needed);
}
return err;
}
从上面的代码,看上去似乎是定义了一个系统调用。这里有一个关于SYSCALL_DEFINE3的不错的blog,提供了思路,可惜排版不好,我就自己找源码摘录下来。位于xxx/linux/kernels/mips-linux-2.6.31/include/linux/syscalls.h
。
Location: xxx/linux/kernels/mips-linux-2.6.31/include/linux/syscalls.h
#define __SC_DECL1(t1, a1) t1 a1
#define __SC_DECL2(t2, a2, ...) t2 a2, __SC_DECL1(__VA_ARGS__)
#define __SC_DECL3(t3, a3, ...) t3 a3, __SC_DECL2(__VA_ARGS__)
#define SYSCALL_DEFINE3(name, ...) SYSCALL_DEFINEx(3, _##name, __VA_ARGS__)
#ifdef CONFIG_FTRACE_SYSCALLS
#define SYSCALL_DEFINEx(x, sname, ...) \
static const char *types_##sname[] = { \
__SC_STR_TDECL##x(__VA_ARGS__) \
}; \
static const char *args_##sname[] = { \
__SC_STR_ADECL##x(__VA_ARGS__) \
}; \
SYSCALL_METADATA(sname, x); \
__SYSCALL_DEFINEx(x, sname, __VA_ARGS__)
#else
#define SYSCALL_DEFINEx(x, sname, ...) \
__SYSCALL_DEFINEx(x, sname, __VA_ARGS__)
#endif
#ifdef CONFIG_HAVE_SYSCALL_WRAPPERS
#define SYSCALL_DEFINE(name) static inline long SYSC_##name
#define __SYSCALL_DEFINEx(x, name, ...) \
asmlinkage long sys##name(__SC_DECL##x(__VA_ARGS__)); \
static inline long SYSC##name(__SC_DECL##x(__VA_ARGS__)); \
asmlinkage long SyS##name(__SC_LONG##x(__VA_ARGS__)) \
{ \
__SC_TEST##x(__VA_ARGS__); \
return (long) SYSC##name(__SC_CAST##x(__VA_ARGS__)); \
} \
SYSCALL_ALIAS(sys##name, SyS##name); \
static inline long SYSC##name(__SC_DECL##x(__VA_ARGS__))
#else /* CONFIG_HAVE_SYSCALL_WRAPPERS */
#define SYSCALL_DEFINE(name) asmlinkage long sys_##name
#define __SYSCALL_DEFINEx(x, name, ...) \
asmlinkage long sys##name(__SC_DECL##x(__VA_ARGS__))
#endif /* CONFIG_HAVE_SYSCALL_WRAPPERS */
照着这个宏一层层剥离:
SYSCALL_DEFINE3(bind, int, fd, struct sockaddr __user *, umyaddr, int, addrlen)
SYSCALL_DEFINEx(3, _bind, __VA_ARGS__)
__SYSCALL_DEFINEx(3, _bind, __VA_ARGS__)
asmlinkage long sys_bind(__SC_DECL3(__VA_ARGS__))
其中 __VA_ARGS__
代表 int, fd, struct sockaddr __user *, umyaddr, int, addrlen
,用__SC_DECL3
逐一展开:
__SC_DECL3(int, fd, struct sockaddr __user *, umyaddr, int, addrlen) --->
int fd, __SC_DECL2(struct sockaddr __user *, umyaddr, int, addrlen) --->
int fd, struct sockaddr __user * umyaddr, __SC_DECL1(int, addrlen) --->
int fd, struct sockaddr __user * umyaddr, int addrlen
那么SYSCALL_DEFINE3(bind, int, fd, struct sockaddr __user *, umyaddr, int, addrlen)
最后表示就是:
asmlinkage long sys_bind(int fd, struct sockaddr __user * umyaddr, int addrlen);
这样解出来的函数其实并不是我们网络编程时候调用的函数bind
,这就很奇怪了,两者是如何联系起来的?这儿我们把疑惑放下,先假设两者是一致的。此时细看一下sys_bind
的实现,注意这几句:
struct sockaddr_storage address;
err = move_addr_to_kernel(umyaddr, addrlen, (struct sockaddr *)&address);
实现:
int move_addr_to_kernel(void __user *uaddr, int ulen, struct sockaddr *kaddr)
{
if (ulen < 0 || ulen > sizeof(struct sockaddr_storage))
return -EINVAL;
if (ulen == 0)
return 0;
if (copy_from_user(kaddr, uaddr, ulen))
return -EFAULT;
return audit_sockaddr(ulen, kaddr);
}
从用户空间拷贝到内核空间时候要检查addrlen
,如果ulen > sizeof(struct sockaddr_storage)
,则会报错。sockaddr_storage
的定义如下:
/* Structure large enough to hold any socket address (with the historical
exception of AF_UNIX). We reserve 128 bytes. */
#define __ss_aligntype unsigned long int
#define _SS_SIZE 128
#define _SS_PADSIZE (_SS_SIZE - (2 * sizeof (__ss_aligntype)))
struct sockaddr_storage
{
__SOCKADDR_COMMON (ss_); /* Address family, etc. */
__ss_aligntype __ss_align; /* Force desired alignment. */
char __ss_padding[_SS_PADSIZE];
};
重点就是:Structure large enough to hold any socket address
,只要是合法的socket address
,就都不会超过它的大小。至此我们可以得出结论,强转指针不会影响原来的结构,毕竟仅仅是传递指针而已。
另外,《UNIX 网络编程 卷1: 套接字联网API》的3.3.2节中也给出了明确的表述:
从应用程序开发人员的观点看,这些通用套接字地址(注: struct sockaddr)结构的唯一用途就是对指定特定于协议的套接字地址结构的指针执行类型强制转换。
...从内核的角度看,使用指向通用套接字地址结构的指针另有原因:内核必须取调用者的指针,把它类型转换为 struct sockaddr * 类型,然后检查其中 sa_family 字段的值以确定这个结构的真是类型。
疑惑2
现在再把前面一个疑惑捡回来,我们编程时候使用的bind
函数和sys_bind
有什么关系?直觉来说,网络编程时调用的bind
函数最后一定调用了sys_bind
函数。
网络编程常用接口的内核实现----sys_bind()
Linux 网络协议栈系列
这个系列文章中提到,socket()
调用的是glibc
里面的__socket()
实现。这里岔开一下,先看一下几个术语名词之间的联系、区别:
关于gcc、glibc和binutils模块之间的关系
这篇文章中提到:glibc是gnu发布的libc库,也即c运行库。glibc是linux系统中最底层的api(应用程序开发接口),几乎其它任何的运行库都会倚赖于glibc。glibc除了封装linux操作系统所提供的系统服务外,它本身也提供了许多其它一些必要功能服务的实现,主要的如下:
(1)string,字符串处理
(2)signal,信号处理
(3)dlfcn,管理共享库的动态加载
(4)direct,文件目录操作
(5)elf,共享库的动态加载器,也即interpreter
(6)iconv,不同字符集的编码转换
(7)inet,socket接口的实现
(8)intl,国际化,也即gettext的实现
(9)io
(10)linuxthreads
(11)locale,本地化
(12)login,虚拟终端设备的管理,及系统的安全访问
(13)malloc,动态内存的分配与管理
(14)nis
(15)stdlib,其它基本功能
libc、glibc和glib的关系
【整理】libc、glibc和glib的关系 原链接
glibc, eglibc和 glib的区别
glibc 和 libc 都是 Linux 下的 C 函数库。libc 是 Linux 下的 ANSI C 函数库;glibc 是 Linux 下的 GUN C 函数库。
ANSI C 函数库是基本的 C 语言函数库,包含了 C 语言最基本的库函数。
GNU C 函数库是一种类似于第三方插件的东西。由于 Linux 是用 C 语言写的,所以 Linux 的一些操作是用 C 语言实现的,因此,GUN 组织开发了一个 C 语言的库 以便让我们更好的利用 C 语言开发基于 Linux 操作系统的程序。 不过现在的不同的 Linux 的发行版本对这两个函数库有不同的处理方法,有的可能已经集成在同一个库里了。
Overview
Any Unix-like operating system needs a C library: the library which defines the ``system calls'' and other basic facilities such as open, malloc, printf, exit...
The GNU C Library is used as the C library in the GNU system and in GNU/Linux systems, as well as many other systems that use Linux as the kernel.
Project Goals
The GNU C Library is primarily designed to be a portable and high performance C library. It follows all relevant standards including ISO C11 and POSIX.1-2008. It is also internationalized and has one of the most complete internationalization interfaces known.
Current Status
The current stable version of glibc is 2.22. See the NEWS file in the glibc sources for more information.
Latest News
2015-08-14: glibc 2.22 released.
2015-02-06: glibc 2.21 released.
2014-09-07: glibc 2.20 released.
2014-02-08: glibc 2.19 released.
2013-08-12: glibc 2.18 released.
History
The history of Unix and various standards determine much of the interface of the C library. In general the GNU C Library supports the ISO C and POSIX standards. We also try to support the features of popular Unix variants (including BSD and System V) when those do not conflict with the standards. Different compatibility modes (selectable when you compile an application) allow the peaceful coexistence of compatibility support for different varieties of Unix.
People
The GNU C Library is currently maintained by a community of developers many of which are listed on the MAINTAINERS page of the project wiki.
Many others have contributed in large amounts as documented in the glibc Contributors.
Thank you to all who have contributed, either in bug reports, or by answering a question, your help is appreciated.
疑惑3
参考1-socket函数在哪个文件定义
参考2-GLIBC 官网
在socket()库函数到系统调用,再到内核中提到__socket()
函数的路径下并没有找到函数实现。猜测可能是glibc
版本变迁造成的迁移?
__attribute__ 之weak,alias属性中提到weak
与alias
属性,同时提到socket
函数在glibc
库中实现方式。
glibc 的实现里面经常用 weak alias。比如它的 socket 函数,在C文件里面你会看到一个 __socket 函数,它几乎什么都没有做,只是设置了一些错误代码,返回些东西而已。在同一个 C 文件里面会再声明一个 __socket 的 weak alias 别名 socket 。实际完成工作的代码通过汇编来实现,在另外的汇编文件里面会有设置系统调用号,执行 sysenter 或者 int 等动作去请求系统调用。以前看 glibc 里面系统调用的实现的时候郁闷过很久,就是那个时候才知道了 weak alias 这个东西。
上面这段描述倒是与socket()库函数到系统调用,再到内核提到的socket
调用路径是一致的。说明早期glibc
库中关于socket
函数实现就是这个思路。
问题来了: 最近版本glibc
[2.19 与 2.22]中相应socket
函数如何实现的?
首先,我们将前面文章中提到的调用关系重复一下:
glibc-2.3.6
- socket()调用如下:
- socket()->__socket():glibc-2.3.6/sysdept/generic/socket.c (weak_alias(name1, name2))
- __socket():glibc-2.3.6/sysdept/unix/sysv/linux/i386/socket.S
- ENTER_KERNEL:
movl $SYS_ify(socketcall), %eax /* System call number in %eax. */
/* Use ## so `socket' is a separate token that might be #define'd. */
movl $P(SOCKOP_,socket), %ebx /* Subcode is first arg to syscall. */
lea 4(%esp), %ecx /* Address of args is 2nd arg. */
/* Do the system call trap. */
ENTER_KERNEL
weak_alias(name1,name2)
为标号name1定义一个弱化名name2。仅当name2没有在任何地方定义时,连接器就会用name1解析name2相关的符号。在文件中定义的标号name1也会同样处理。
glibc-2.19
- socket()->__socket():glibc-2.19/socket/socket.c
/* Create a new socket of type TYPE in domain DOMAIN, using
protocol PROTOCOL. If PROTOCOL is zero, one is chosen automatically.
Returns a file descriptor for the new socket, or -1 for errors. */
int
__socket (domain, type, protocol)
int domain;
int type;
int protocol;
{
__set_errno (ENOSYS);
return -1;
}
weak_alias (__socket, socket)
stub_warning (socket)
- __socket():glibc-2.19/sysdeps/unix/sysv/linux/i386/socket.S
/* Save registers. */
movl %ebx, %edx
cfi_register (3, 2)
movl $SYS_ify(socketcall), %eax /* System call number in %eax. */
/* Use ## so `socket' is a separate token that might be #define'd. */
movl $P(SOCKOP_,socket), %ebx /* Subcode is first arg to syscall. */
lea 4(%esp), %ecx /* Address of args is 2nd arg. */
/* Do the system call trap. */
ENTER_KERNEL
这说明直到2.19,glibc
的socket
函数调用方式还是一致的。
glibc-2.22
- socket()->__socket():glibc-2.22/socket/socket.c
weak_alias
的函数依然在这里定义。 - __socket():glibc-2.22/sysdeps/unix/sysv/linux/socket.c
int
__socket (int fd, int type, int domain)
{
return SOCKETCALL (socket, fd, type, domain);
}
libc_hidden_def (__socket)
weak_alias (__socket, socket)
- SOCKETCALL:glibc-2.22/sysdeps/unix/sysv/linux/socketcall.h
#define __SOCKETCALL3(name, a1, a2, a3) \
INLINE_SYSCALL (socketcall, 2, name, \
((long int [3]) { (long int) (a1), (long int) (a2), (long int) (a3) }))
#define __SOCKETCALL_NARGS_X(a,b,c,d,e,f,g,h,n,...) n
#define __SOCKETCALL_NARGS(...) \
__SOCKETCALL_NARGS_X (__VA_ARGS__,7,6,5,4,3,2,1,0,)
#define __SOCKETCALL_CONCAT_X(a,b) a##b
#define __SOCKETCALL_CONCAT(a,b) __SOCKETCALL_CONCAT_X (a, b)
#define __SOCKETCALL_DISP(b,...) \
__SOCKETCALL_CONCAT (b,__SOCKETCALL_NARGS(__VA_ARGS__))(__VA_ARGS__)
#define __SOCKETCALL(...) __SOCKETCALL_DISP (__SOCKETCALL, __VA_ARGS__)
#define SOCKETCALL(name, args...) \
({ \
long int sc_ret = __SOCKETCALL (SOCKOP_##name, args); \
sc_ret; \
})
OK,这段鬼代码翻译一下就是
SOCKETCALL (socket, fd, type, domain); --->
__SOCKETCALL (SOCKOP_socket, fd, type, domain); --->
__SOCKETCALL_DISP (__SOCKETCALL,SOCKOP_socket, fd, type, domain); --->
__SOCKETCALL_CONCAT (__SOCKETCALL,__SOCKETCALL_NARGS(SOCKOP_socket, fd, type, domain))(SOCKOP_socket, fd, type, domain); --->
__SOCKETCALL_CONCAT_X (__SOCKETCALL, __SOCKETCALL_NARGS_X(SOCKOP_socket, fd, type, domain,7,6,5,4,3,2,1,0,))(SOCKOP_socket, fd, type, domain); --->
__SOCKETCALL_CONCAT_X (__SOCKETCALL, 3)(SOCKOP_socket, fd, type, domain);
__SOCKETCALL3(SOCKOP_socket, fd, type, domain); --->
INLINE_SYSCALL (socketcall, 2, SOCKOP_socket, ((long int [3]) { (long int) (fd), (long int) (type), (long int) (domain) }))
这INLINE_SYSCALL
又是什么鬼东西?在代码里面搜了搜,还比较多,其中:glibc-2.22/sysdeps/unix/sysdep.h里面的定义比较靠谱,毕竟是与上面的代码有共同的祖先目录。
/* Wrappers around system calls should normally inline the system call code.
But sometimes it is not possible or implemented and we use this code. */
#ifndef INLINE_SYSCALL
#define INLINE_SYSCALL(name, nr, args...) __syscall_##name (args)
#endif
上面说:包裹系统调用的函数一般会内联系统调用的代码。从上面的宏定义看,我们的INLINE_SYSCALL
是解不开的。那么这些内联代码应该是在glibc-2.22/sysdeps/unix/sysv/linux/下的子目录里面,各个子目录是不同的硬件平台:
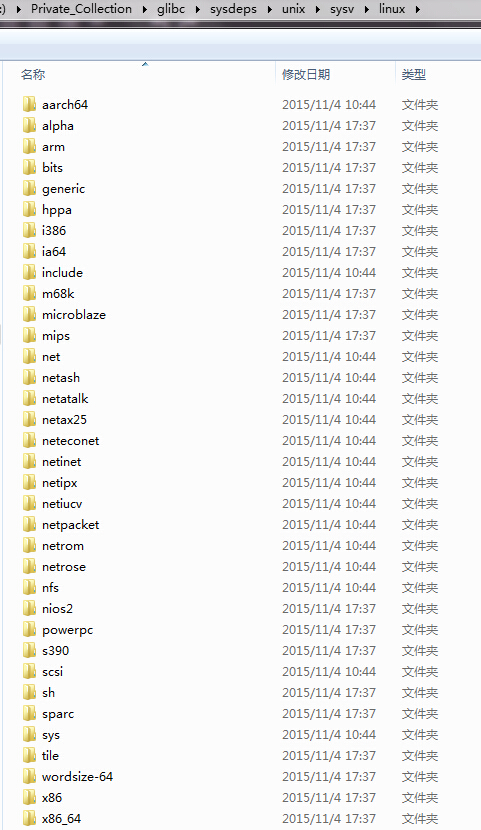
以i386为例,看一下:glibc-2.22/sysdeps/unix/sysv/linux/i386/sysdep.h
#undef INLINE_SYSCALL
#define INLINE_SYSCALL(name, nr, args...) \
({ \
unsigned int resultvar = INTERNAL_SYSCALL (name, , nr, args); \
if (__glibc_unlikely (INTERNAL_SYSCALL_ERROR_P (resultvar, ))) \
{ \
__set_errno (INTERNAL_SYSCALL_ERRNO (resultvar, )); \
resultvar = 0xffffffff; \
} \
(int) resultvar; })
INLINE_SYSCALL (socketcall, 2, SOCKOP_socket, ((long int [3]) { (long int) (fd), (long int) (type), (long int) (domain) })) --->
({
unsigned int resultvar = INTERNAL_SYSCALL (socketcall, , 2, SOCKOP_socket, ((long int [3]) { (long int) (fd), (long int) (type), (long int) (domain) }));
if (__glibc_unlikely (INTERNAL_SYSCALL_ERROR_P (resultvar, )))
{
__set_errno (INTERNAL_SYSCALL_ERRNO (resultvar, ));
resultvar = 0xffffffff;
}
(int) resultvar;
})
其中,
#define INTERNAL_SYSCALL(name, err, nr, args...) \
({ \
register unsigned int resultvar; \
INTERNAL_SYSCALL_MAIN_##nr (name, err, args); \
(int) resultvar; })
#define INTERNAL_SYSCALL_MAIN_2(name, err, args...) \
INTERNAL_SYSCALL_MAIN_INLINE(name, err, 2, args)
# define INTERNAL_SYSCALL_MAIN_INLINE(name, err, nr, args...) \
EXTRAVAR_##nr \
asm volatile ( \
LOADARGS_##nr \
"movl %1, %%eax\n\t" \
"call *%%gs:%P2\n\t" \
RESTOREARGS_##nr \
: "=a" (resultvar) \
: "i" (__NR_##name), "i" (offsetof (tcbhead_t, sysinfo)) \
ASMFMT_##nr(args) : "memory", "cc")
INTERNAL_SYSCALL (socketcall, , 2, SOCKOP_socket, ((long int [3]) { (long int) (fd), (long int) (type), (long int) (domain) })) --->
({
register unsigned int resultvar;
INTERNAL_SYSCALL_MAIN_2 (socketcall, err, SOCKOP_socket, ((long int [3]) { (long int) (fd), (long int) (type), (long int) (domain) }));
(int) resultvar;
})--->
({
register unsigned int resultvar;
INTERNAL_SYSCALL_MAIN_INLINE (socketcall, err, 2, SOCKOP_socket, ((long int [3]) { (long int) (fd), (long int) (type), (long int) (domain) }));
(int) resultvar;
})--->
卧槽,到汇编了,我搞不下去了。
尼玛,2.22版本太虐心了,我还是参考2.19吧。那个应该比较好分析一点。
分析glibc-2.19 socket
函数
__socket():glibc-2.19/sysdeps/unix/sysv/linux/i386/socket.S
#define P(a, b) P2(a, b)
#define P2(a, b) a##b
...
/* Save registers. */
movl %ebx, %edx
cfi_register (3, 2)
movl $SYS_ify(socketcall), %eax /* System call number in %eax. */
/* Use ## so `socket' is a separate token that might be #define'd. */
movl $P(SOCKOP_,socket), %ebx /* Subcode is first arg to syscall. */
lea 4(%esp), %ecx /* Address of args is 2nd arg. */
/* Do the system call trap. */
ENTER_KERNEL
其中,
SYS_ify:glibc-2.19/sysdeps/unix/sysv/linux/i386/sysdep.h
#undef SYS_ify
#define SYS_ify(syscall_name) __NR_##syscall_name
因此,
/* Save registers. */
movl %ebx, %edx
cfi_register (3, 2)
movl $__NR_socketcall, %eax /* System call number in %eax. */
/* Use ## so `socket' is a separate token that might be #define'd. */
movl $SOCKOP_socket, %ebx /* Subcode is first arg to syscall. */
lea 4(%esp), %ecx /* Address of args is 2nd arg. */
/* Do the system call trap. */
ENTER_KERNEL
其中,
SOCKOP_socket : glibc-2.19/sysdeps/unix/sysv/linux/socketcall.h
#define SOCKOP_socket 1
__NR_socketcall
的定义也找不到,从名字看,应该是一个数字(number
)。暂时不管,这里面最重要的就是ENTER_KERNEL
:glibc-2.19/sysdeps/unix/sysv/linux/i386/sysdep.h
/* The original calling convention for system calls on Linux/i386 is
to use int $0x80. */
#ifdef I386_USE_SYSENTER
# ifdef SHARED
# define ENTER_KERNEL call *%gs:SYSINFO_OFFSET
# else
# define ENTER_KERNEL call *_dl_sysinfo
# endif
#else
# define ENTER_KERNEL int $0x80
#endif
Linux/i386平台上系统调用的原始调用惯例是使用int $0x80
。稍微熟悉i386
处理器的应该知道,这条汇编语句是i386
的软中断指令。
在许多汇编中常有int $0x80,int $0x80究竟是个什么样的软中断?
Linux用int $80来实现系统调用异常 .这一指令使用中断/异常向量号128(即16进制的80)将控制权转移给内核。为达到在使用系统调用时不必用机器指令编程,在标准的C语言库中为每一系统调用提供了一段短的子程序,完成机器代码的编程工作。事实上,机器代码段非常简短。它所要做的工作只是将送给系统调用的参数加载到CPU寄存器中,接着执行int $0x80指令。然后运行系统调用,系统调用的返回值将送入CPU的一个寄存器中,标准的库子程序取得这一返回值,并将它送回用户程序.
一般现在的核心有190个左右的系统调用,可以看/usr/src/linux/代码目录下的
init/main.c
arch/i386/kernel/traps.c
arch/i386/kernel/entry.S
arch/i386/kernel/irq.h
include/asm-386/unistd.h
总结一下socket.S
里面汇编代码的意思,参考链接:
- 将系统调用号
__NR_socketcall
存入eax
寄存器 - 将系统调用子码
SOCKOP_socket
存入ebx
寄存器,子码是syscall
的第一个参数。 - 取出栈指针寄存器
esp
的值加4
,然后存入ecx
寄存器。参数地址是第二个参数? - 靠软中断(也称陷阱)进入内核,内核负责处理系统调用。
我们知道函数调用要遵循调用惯例,也就是所谓的ABI,如cdecl规范【所谓cdecl调用由调用者负责恢复栈,调用者负责清理的只是入栈的参数,被调用函数自己的堆栈空间返回时自己已经清除】。此处似乎与函数调用无关,需要深入研究一下。
TO BE CONTINUED
再了解一下Linux网络协议栈架构:
Linux 网络栈剖析

网友评论